By freezing the perovskites in a glass host matrix, we can trap the active phase and improve stability to unlock the full potential of the next generation of photovoltaic and electronic devices.
Since ancient times, glass has illuminated the world with its exquisite elegance and splendor. Whilst being known for its clarity and transparency, the underlying structure remains opaque and elusive which has intrigued scientists for centuries. The curiosity was sparked by the rumor that old stained glass appeared thicker at the bottom. This implied that glass was like a liquid and flowed slowly, creating the enigmatic question of whether glass was a solid or a liquid.
Based on the transparent allure of glass, we draw the parallel of glass being a liquid frozen in time. Yet, other scientists contended that the rigidity and stability of glass can only mean it is a solid. Eventually, a consensus was reached to label glass as a “frozen liquid”. Glass embodies the amorphous and disordered structure of liquids, and during rapid cooling, the atoms or molecules are “frozen” into a rigid solid state, capturing the disordered arrangement of the liquid-like structure.
Traditionally, glasses are categorised into three families: organic (polymers), inorganic (oxides, oxynitrides, and chalocogenides) and metallic glasses. In 2015, the Bennett group at the University of Cambridge introduced a new category of glass, the hybrid inorganic-organic glass. [1] This exciting discovery expands the chemical diversity and materials properties available for exploration.
Perovskites have captured our attention due to their exceptional light absorption and cost-effective processing, making them promising for applications in photovoltaics and electronic devices. Unfortunately, perovskites are notorious for their poor stability. By encapsulating perovskites within a hybrid glass matrix, we can effectively shield them from degradation by light, heat, and solvents. [2]
Moreover, the glass matrix acts to stabilise the active perovskite phase. Perovskites can exist in two different phases: yellow and black. The black phase is desired for its exceptional light absorption, efficient charge transport, and desirable carrier mobility, whereas the yellow phase is inactive. However, under normal conditions, the inactive yellow phase is thermodynamically favoured. Therefore, we must strategically develop methods to confine and stabilise the perovskite black phase. By embedding it within a glass matrix composite and utilising interfacial interactions, we can elegantly freeze the perovskite into the active black phase.
To investigate the complex interactions between the glass-perovskite composites, we will employ bespoke scanning electron microscopy. This cutting-edge technique allows us to peer through the looking glass and probe the nanoscale domains and interfacial interactions. By continuously scanning across each pixel, we can create a map that details the abrupt interface between the amorphous glass and crystalline perovskite. The outstanding spatial resolution offered by this state-of-the-art technique, pioneered by the University of Cambridge, is unparalleled in mapping such intricate nanostructures. [3]
Through this research, we aim to shed light on the black-yellow perovskite phase transition and gain a deeper understanding of the interfacial interactions at play. This knowledge holds great potential for advancing the development of perovskite-based technologies and unlocking their full capabilities.
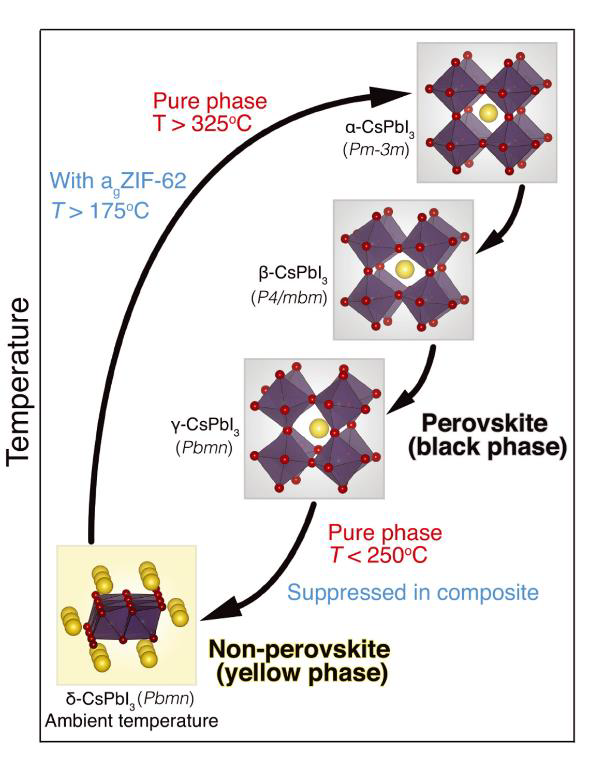
Phase transition of perovskites in its pure phase and encapsulated within the composite. Ref [2]
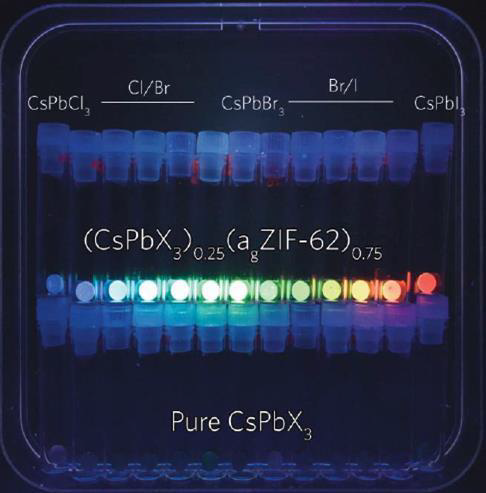
References:
- Bennett, T.D., et al., Hybrid glasses from strong and fragile metal-organic framework liquids. Nature Communications, 2015. 6(1): p. 8079.
- Hou, J., et al., Liquid-phase sintering of lead halide perovskites and metal-organic framework glasses. Science, 2021. 374(6567): p. 621-625.
- Laulainen, J.E., et al., Mapping short-range order at the nanoscale in metal–organic framework and inorganic glass composites. Nanoscale, 2022. 14(44): p. 16524-16535.
NanoDTC PhD Student, c2022